The potential of physiological monitoring technologies in esports
by Aaron Koshy*, George Mathew KoshyEmail: a.koshy@nhs.net
Received: 26 Aug 2020 / Published: 04 Oct 2020
Abstract
Esports is a rapidly growing sector with increasing investment and large-scale international tournaments offering significant prize pools. This has led to a greater focus on individual and team performance with factors such as communication and team intelligence identified as important for success. Over a similar time period, monitoring technologies have become commonplace with clinical grade assessment available in a number of parameters that have evidenced utility. Despite this, physiological monitoring is not routine within esports. In this scoping review, we explore the options available for esports organisations and the potential barriers to implementation. A model for effective integration of these tools has been devised to mitigate the wastage of resources and increase collaboration between academia, healthcare professionals esports organisations and industry. We expect that in the near future, monitoring devices will become an essential part of performance assessment, enhancement, injury prevention and general management for players and esports teams.
Highlights
- There are multiple monitoring devices available that can measure physiological parameters of esports players without noticeably hindering their performance.
- Limitations and barriers exist within current physiological monitoring devices that must be considered before implementation within the esports environment.
- We have devised a model to maximise value and safety in integrating physiological monitoring technologies within an esports organisation.
Background
Esports is the organised competition of players or teams within video gaming. It is increasingly focused on performance and sporting success with many professional players receiving a similar level of training to elite traditional sports athletes [1]. Esports has steadily entered mainstream entertainment in the last decade and revolves around players or teams working to beat their counterparts often in a series of objectives. Popular esports games such as League of Legends or Counter Strike: Global Offensive enjoy viewership in the many tens of millions at their premier live tournaments with figures that rival traditional sports [2]. Revenue streams into esports have also ballooned, bypassing $1 billion in 2019 [3]. This has trickled down to major increases in spending within professional teams on players, coaching and facilities to improve performance. The staff that support the players have also expanded to include multiple managers, coaches, physiotherapists, sports psychologists and data analysts.
A number of studies have already scratched the surface of performance determinants within esports. Unsurprisingly for team games, communication and collective intelligence are shown to be predictors of success [4]. Teamwork is critical at a professional and casual level within esports [5]. This expands to the point where players may not be selected or even dropped from a team if there are weaknesses in these domains. Indeed, these qualities may translate into the game through measurable and significant outcomes predictive of success, such as fewer deaths and better map control [6]. For example, fewer deaths mean that the team generally has a higher chance of winning decisive battles through higher damage or player numbers whereas map control often forces the opposing team into disadvantageous positions.
With the rise of esports, consumer monitoring technologies and clinical grade assessments have become more accessible. Smart watches and fitness trackers have a large market value that is forecast to grow to $62 billion by 2023 [7]. As adoption increases, demand for functionality has broadened from heart rate monitoring to heart rate variability and sleep analysis. This shift also highlights the interest beyond non-physiological parameters, from location and movement to physiological measurements (which directly or indirectly measure body function), such as heart rate and temperature. It was identified as early as 1998 that non-invasive devices can not only consistently display meaningful physiological data, but also serve in situations where traditional measuring tools are not viable [8]. These clinical tools are now routinely used in a variety of situations such as monitoring patients for signs of deterioration, in the research setting as predictors of outcome or as a response to intervention. The technologies are also employed in a number of occupations and sports with heart rate monitoring shown to be an effective marker of stress in pilots during training [9]. The military has also embraced assessment technology as a method of improving performance by collecting information on soldier fitness, alertness and their psychological state [10]. Formula One racing could be one of the earliest adopters of monitoring technologies in sports due to the increasing awareness of the physical and mental strain applied on the drivers [11, 12].
As the rewards for success increase alongside knowledge of the stressors within esports, we expect monitoring devices to play a key role in identifying and improving outcomes. This article discusses the potential value of physiological monitoring within esports, the current tools that could be utilised, the barriers that must be overcome to ensure full implementation and proposes a model for application.
Methods
Monitoring devices can assess a plethora of parameters from heart rate to skin temperature, many of which could vary during competitive esports games due to the associated stress, complex decision making, teamwork and even duration of activity. For this scoping review, we undertook a structured PubMed, ResearchGate and Google search from 20-26th May 2020 to identify articles published from 1946 to May 2020 including the following search terms: Esports, e-sports, gaming, video gaming, computer gaming, competitive gaming, monitoring, wearables, portable devices, cardiac output (CO), heart rate (HR), heart rate variability (HRV), blood pressure (BP), oxygen saturation (SO2) and non-invasive. Grey literature was also searched using Google search and Google Scholar. The abstracts were reviewed and considered for inclusion based on the description and relevance; specifically, devices were included in this review if they could be utilised in the esports setting during training or competitive matches without causing obvious hindrance. Technologies were excluded if they only provide a single snapshot reading, fail to validate findings against the gold standard for the relevant parameter, or were not commercially available at the time of searching.
Viable monitoring devices and measuring parameters in Esports
One of the most commonly utilised portable technologies are smartwatches and fitness trackers. These devices have grown in variety with increasing functionality over successive generations. It is now common for physiological parameters such as HR, temperature and activity levels to be measured, often with the aim of improving health outcomes ranging from self-management of diabetes to seizure monitoring [13]. However, despite high levels of investment, few of these devices have managed to reach clinical grade accuracy or reliability [14]. Similarly, sleep trackers are increasingly common place and are a cost effective tool for monitoring sleep, though they appear to vary in reliability [15, 16]. In the context of esports, these devices could be ideal in the setting of a gaming house; an arrangement in which players cohabit a tailored facility with access to high speed internet and quality computing equipment. In this environment, many factors are often more controlled such as sleep and activity cycles.
Clinically focused wearables conversely have generally completed longer periods of device evolution with more stringent standards for approval and application. These technologies are often based on known physiological principles adapted for utility under controlled conditions. Whilst this makes the readings more reliable, the devices themselves are more difficult to exploit outside of their limited tested environment. Furthermore, a lack of awareness and the generally higher cost make these options less accessible. We believe that both general consumer and clinically focused technologies offer value to esports. A list of technologies has been generated (table 1) that are validated against the gold standard, applicable in the esports environment and currently available to purchase. The devices range from a monitor placed on the wrist to sensors distributed within clothing. Importantly, these devices should in theory allow the player to compete as normal without hindrance. The diversity of tools enables players and teams to work in a minimally restrictive manner whilst maximising analytical potential with scope to combine technologies, expanding monitoring capability further. Notably, portable devices frequently utilise technologies that can derive multiple physiological parameters such as bioimpedance (a measure of electrical resistance through the body) to obtain heart rate and respiratory rate with reasonable levels of accuracy [17, 18]. This is useful as playing video games can increase psychophysiological arousal manifesting in parameters such as heart rate change suggesting that the cognitive and subsequent physical load can be significant [19]. The emergence of miniaturisation, new scientific techniques and consumer demand enables a variety of physiological parameters to be measured, many of which have associations to exercise and mental activities:
Cardiovascular
HR is one of the most commonly assessed physiological parameters and varies with both physical and cognitive exertion [20]. It is often treated as a surrogate for sympathetic activity and can be helpful to assess stress. Specific changes in heart rate are broadly termed as HRV. This is an umbrella term for changes found in sections of the electrical cardiac cycle and has been frequently associated with exercise and even mortality in patient groups [21, 22]. HRV appears to vary within video gaming and may be related to a variety of factors such as the activities within the game, the content shown or difficulty of the task [23]. Furthermore, work by Lee et al [24] suggests that HRV will also differ based on the background of the player. Participants fulfilling the criteria for internet gaming disorder more often have suppression of high frequency HRV, a phenomenon that is associated with parasympathetic inhibition and emotions such as stress or anxiety [25]. Additionally, the gold standard for analysing the complete electrical cycle, the 12-lead electrocardiogram (ECG or EKG) can be employed. ECGs are extremely effective at identifying the current HR and capturing on-going arrhythmias. Unfortunately, this is rarely practical outside of the hospital setting, especially in the context of body movement. Portable devices with ECG capability are common and generally utilise 1-3 leads making it viable for general monitoring but are rarely used in the diagnostic setting; with acceptable accuracy only present in specific scenarios such as screening for arrhythmias like atrial fibrillation [26]. Cardiac anatomy and function also relate to exercise performance [27]. The stroke volume (SV) is the volume of blood pumped from the heart per beat. The SV can be multiplied by the HR to derive the cardiac output (CO) which is the volume of blood pumped by the heart in one minute and is linked to exercise capacity. Notably, the SV and CO have been shown to increase with higher cognitive load and exercise [28, 29].
Respiratory
Breathing changes are expected during times of physical or mental stress [30]. Two common parameters are the respiratory rate (RR), which is the number of breaths taken in one minute and minute ventilation; the volume of air that is inhaled or exhaled in one minute. Activities that require higher concentration or are more cognitively demanding result in a higher RR and minute ventilation [30]. Furthermore, difficult mental tasks are associated with reductions in oxygen saturation (SO2) which is the proportion of oxygenated haemoglobin to total haemoglobin in the blood [31].
Other
Skin temperature is frequently found on monitoring devices as historically, temperature has been a key parameter of health. The temperature of the skin is also relatively easy to measure with modern sensors which in turn enables an accurate estimation of the more clinically valuable parameter, core body temperature [32]. Recently, skin temperature has been identified as a potentially significant factor in aerobic performance suggesting intrinsic value of this measure [33]. The expansion of smartphone use has also led to the development of movement sensors such as accelerometers which are similarly commonplace in technologies such as fitness watches. Despite their popularity, these tools are generally poorly validated with variable accuracy and should be assessed on a case by case basis [33, 34].
Overall, the physiological parameters of players must be carefully managed in esports with input from academic, healthcare or industry partners to ensure a high-quality methodology and analysis, thus enabling a meaningful interpretation. Without such oversight there would be a higher risk of false attribution or correlation, leading to wastage of resources or potentially damaging organisational decisions.
Technology name | Description | Device location | Parameters assessed |
---|---|---|---|
Bodyguardian*[35] | Wireless monitor | Placed over chest | HR, ECG, RR and movement |
BPro [36] | Wireless BP (without cuff) monitor | Placed on wrist | BP |
Equivital EQ02 Lifemonitor [37] | Semi-wireless monitor | Electrodes and device placed on chest | HR, HRV, ECG, RR, Skin temperature and movement |
Hexoskin [38] | Wireless monitors within a shirt | Placed on chest | HR, HRV, ECG, RR, minute ventilation, tidal volume and movement |
Ithlete with ECG monitor [39] | Belt with attached wireless monitor | Placed on chest | HR and HRV |
Polar heart rate sensor [40] | Belt with attached wireless monitor | Placed on chest | HR and HRV |
NICOM [41] | Wired monitor | Electrodes placed on chest with wired device placed 1m away | HR, SV and CO |
Qardiocore [42] | Belt with attached wireless monitor | Placed on chest | ECG, HR, HRV, Skin temperature, respiratory rate and movement |
Oxitone 1000M [43] | Wireless monitor | Placed on wrist | HR and SO2 |
Somnotouch [44] | Semi-wireless monitor | Placed on arm ± hand | BP, SO2 and ECG |
Suunto smart sensor [45] | Belt with attached wireless monitor | Placed over chest | HR and HRV |
Zephyr BioPatch HP [46] | Belt with attached wireless monitor | Placed over chest | ECG, Body temperature, HR, HRV, RR and movement |
Zio XT [47] | Wireless monitor | Placed over chest | ECG, HR and HRV |
*Successful validation of some parameters.
Table 1 – Commercially available monitoring technologies (excluding sleep monitors) that are validated against the gold standard and feasible for esports athletes during performance. Validation studies have been referenced with the technology name. BP – Blood pressure, CO – Cardiac output, HR – Heart rate, HRV – Heart rate variability, NA – Not applicable, RR – Respiratory rate, SO2 – Oxygen saturation, SV – Stroke volume.
Barriers to monitoring technology uptake in esports
Utilising physiological monitoring devices must be considered with care. Performing at the highest level of esports has multiple requirements including fine hand control, mental concentration and teamwork. Many commercially available tools would fail at the most practical level of not overly impeding these critical areas. For example, many BP monitors rely on an inflatable cuff to control blood flow and derive a value. Furthermore, parameters such as BP and HR are largely redundant in isolation, with the identification of trends being more important. This leads to a situation where obtaining the physiological values may hinder performance and even alter the measurements themselves.
Regardless of the complexity involved in the implementation of a monitoring technology, the device manufacturers may need to be involved at an early stage to ensure that the esports environment; with the various potential confounders such as high density of digital equipment and alternating activity regimen, would not significantly disturb the accuracy or reliability of the assessment tool [48]. An example would be the smartwatch and wearables market. These are frequently found with optical heart rate sensors that are accurate at rest but are often less reliable during exertion or HR changes; undoubtedly a consideration in competitive gaming which may be compounded by player discomfort when competing whilst wearing the device [49].
The wearables market is rapidly evolving with previously dominant or successful products ending up unused or obsolete. Indeed many of the technologies published in previous reviews are no longer available for a myriad of reasons, such as lack of efficacy post analysis or funding issues [50, 51]. This emphasises the value of a trial period, expert guidance and empirical research.
The introduction of monitoring devices into competitive games will also need to be evaluated by the game publisher or tournament organisers. These tools could be misused to gain an unfair competitive advantage or, indeed inadvertently cause communication issues with essential equipment. For example, increasing the density of wireless communication pathways can cause starvation of data transfer across commonly used wireless protocols [52].
Finally, the cost of implementation may be prohibitive for some esports organisations. Some of the devices in Table 1 have significant capital costs which may increase to obtain extended support for additional monitoring and impact. As the translatable gains are yet to be evidenced, some organisations may have to follow successful implementation from competitors instead of pioneering themselves.
Future of physiological monitoring devices in esports
Esports has grown with the rise of available technologies making the concept of live monitoring players during training and matches not only viable but potentially game changing. Our research suggests that there is increasing overlap between clinically focused devices and general consumer wearables with clinical grade measuring capability, making this an exciting area for further study. This is a novel route for esports players and staff thus we have designed a model for the implementation of monitoring devices (figure 1). With a plethora of options that are likely to increase further, it will be difficult for teams to select the ideal device or composition of tools. Along with collaboration, it would be expected that devices are trialed with a clear methodology and set expectations to avoid poor outcomes and maximise value.
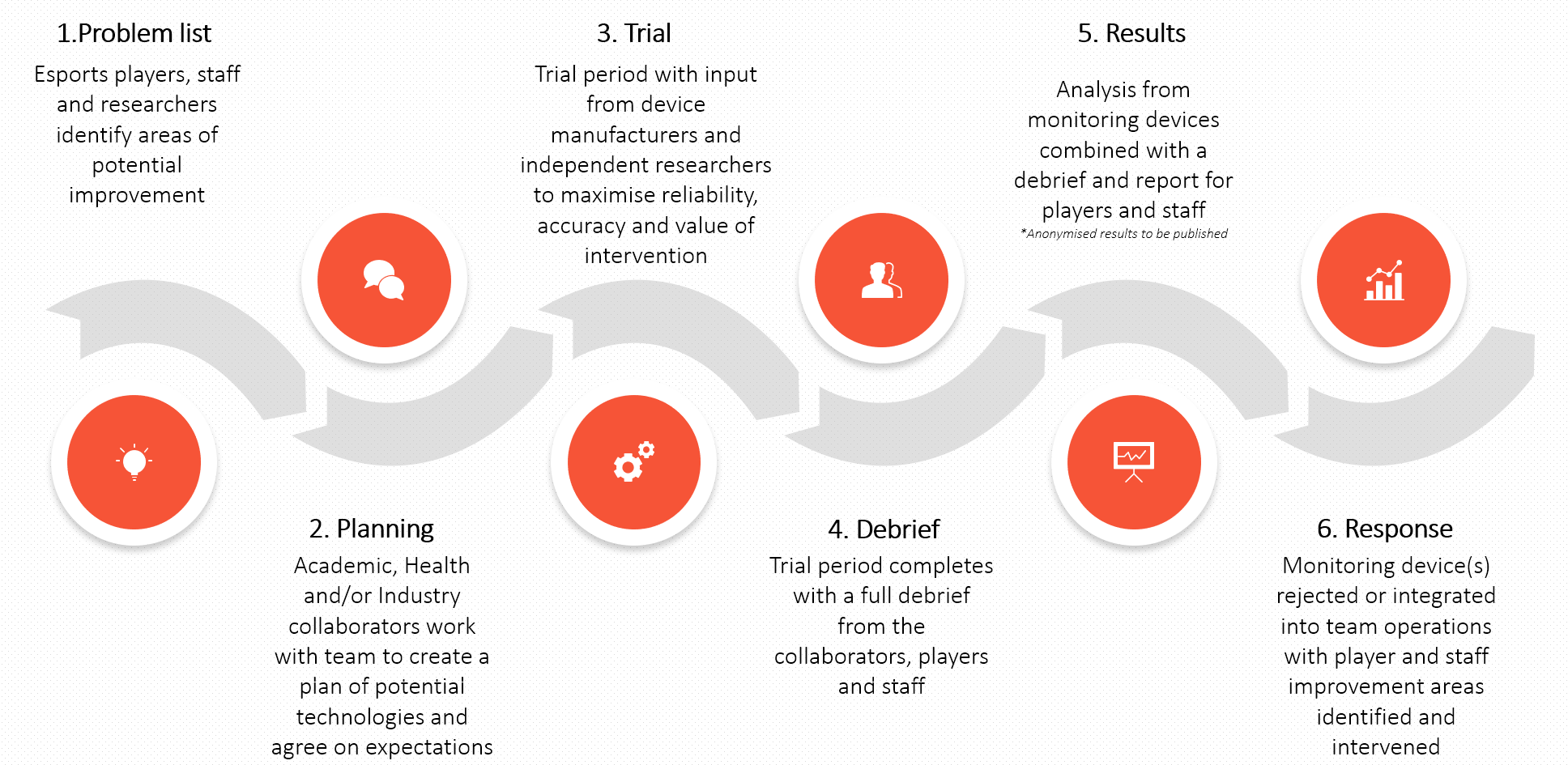
Figure 1 – Proposed model for integration of monitoring devices into Esports teams.
We expect monitoring to extend outwards from training to the live stage. Indeed, DreamHack 2011 had esports players compete with live heart rate monitoring [53]. Whilst this was short-lived, it gives an insight into the potential of monitoring devices and may also provide another exciting dimension for the spectator. The integration of monitoring devices into esports training and competitive games may represent a paradigm shift in performance for players and teams. The objective measures could explain certain performance issues or clutch decision making. Physiological parameters can also aid the physical and sports psychology training that is relatively common within teams. Using a similar model, Sousa et al [54] found that haemodynamic changes are present during esports activity with a modified effect depending on the type of game played. Eye tracking and facial recognition are other areas with advances in software enabling automated analysis to take place rapidly and accurately, leading to the possibility of a gold standard in the near future [55]. This is particularly exciting for esports as there is likely to be a high visual load during activity which through assessment could improve performance, especially when used in conjunction with other technologies [56]. If carefully implemented, monitoring devices could lead to further rewards such as team modelling enabling fanciful concepts such as ideal team role allocation and optimised professional player acquisition based on objective data. The novel application of technology is also showing utility in improving performance. Minor electrical stimulation of the frontal cortex through a forehead patch is associated with a significant improvement in working memory [57]. Alternatively, virtual reality has evidenced value in the acquisition and retention of skills (across physical and psychological domains) within traditional sports such as rowing [58]. Esports as a burgeoning sector is well placed to leverage these advancements to improve the quality of training and competition whilst developing understanding of these applications further; indeed, potentially resulting in the production of esports specific devices. As revenue and mainstream demand increases, we expect partnerships between esports organisations, academia, healthcare and industry to dramatically increase in order to achieve these overlapping areas of interest.
Conclusion
There are multiple market ready technologies that are viable for esports players and teams to integrate into their training and competitive games. This is expected to be a key step in improving performance in esports under careful management and collaboration with academia, healthcare professionals and industry partners.
Conflict of interest
The authors declare they do not have anything to disclose regarding a conflict of interest with respect to this article.
References
- Jenny SE, Manning RD, Keiper MC, Olrich TW. Virtual(ly) athletes: Where esports fit within the definition of “sport”. Quest. 2017;69(1):1-18.
- Sachs G. The world of games: Esports from wild west to mainstream. 2018.
- Newzoo. Global esports market report. 2019.
- Kim YJ, Engel D, Woolley A, Lin J, McArthur N, Malone T. What makes a strong team?: Using collective intelligence to predict team performance in league of legends. Proceedings of the 2017 ACM Conference on Computer Supported Cooperative Work and Social Computing. 2017:2316-29.
- Freeman G, Wohn D. Social support in esports: Building emotional and esteem support from instrumental support interactions in a highly competitive environment2017. 435-47 p.
- Nascimento Junior FFd, Da Costa Melo AS, Da Costa IB, Marinho LB. Profiling successful team behaviors in league of legends2017. 261-8 p.
- Research AM. Fitness trackers market - global opportunity analysis and industry forecast, 2017-2023. 2018.
- Shoemaker WC, Belzberg H, Wo CC, Milzman DP, Pasquale MD, Baga L, et al. Multicenter study of noninvasive monitoring systems as alternatives to invasive monitoring of acutely ill emergency patients. Chest. 1998;114(6):1643-52.
- Regula M, Socha V, Kutílek P, Socha L, Hána K, Hanáková L, et al., editors. Study of heart rate as the main stress indicator in aircraft pilots. Proceedings of the 16th International Conference on Mechatronics - Mechatronika 2014; 2014 3-5 Dec. 2014.
- Friedl KE. Military applications of soldier physiological monitoring. Journal of Science and Medicine in Sport. 2018;21(11):1147-53.
- Brown J, Stanton N, Revell K. A review of the physical, psychological and psychophysiological effects of motorsport on drivers and their potential influences on cockpit interface design. 2019. p. 514-22.
- Reid MB, Lightfoot JT. The physiology of auto racing. Medicine & Science in Sports & Exercise. 2019;51(12):2548-62.
- Reeder B, David A. Health at hand: A systematic review of smart watch uses for health and wellness. Journal of Biomedical Informatics. 2016;63:269-76.
- Feehan LM, Geldman J, Sayre EC, Park C, Ezzat AM, Yoo JY, et al. Accuracy of fitbit devices: Systematic review and narrative syntheses of quantitative data. JMIR mHealth and uHealth. 2018;6(8):e10527-e.
- Evenson KR, Goto MM, Furberg RD. Systematic review of the validity and reliability of consumer-wearable activity trackers. International Journal of Behavioral Nutrition and Physical Activity. 2015;12(1):159.
- Guillodo E, Lemey C, Simmonet M, Ropars J, Berrouiguet S. Sleep monitoring and wearables : A systematic review of clinical trials and future applications (preprint)2018.
- Seppä V-P, Väisänen J, Kauppinen P, Malmivuo J, Hyttinen J, editors. Measuring respirational parameters with a wearable bioimpedance device2007; Berlin, Heidelberg: Springer Berlin Heidelberg.
- Diaz DH, Casas O, Pallas-Areny R. Heart rate detection from single-foot plantar bioimpedance measurements in a weighing scale. Conf Proc IEEE Eng Med Biol Soc. 2010;2010:6489-92.
- Drachen A, Nacke LE, Yannakakis G, Pedersen AL. Correlation between heart rate, electrodermal activity and player experience in first-person shooter games. Proceedings of the 5th ACM SIGGRAPH Symposium on Video Games; Los Angeles, California: Association for Computing Machinery; 2010. p. 49–54.
- Scholey AB, Moss MC, Neave N, Wesnes K. Cognitive performance, hyperoxia, and heart rate following oxygen administration in healthy young adults. Physiol Behav. 1999;67(5):783-9.
- Michael S, Graham KS, Davis GMO. Cardiac autonomic responses during exercise and post-exercise recovery using heart rate variability and systolic time intervals-a review. Frontiers in physiology. 2017;8:301-.
- Hillebrand S, Gast KB, de Mutsert R, Swenne CA, Jukema JW, Middeldorp S, et al. Heart rate variability and first cardiovascular event in populations without known cardiovascular disease: Meta-analysis and dose–response meta-regression. EP Europace. 2013;15(5):742-9.
- Porter AM, Goolkasian P. Video games and stress: How stress appraisals and game content affect cardiovascular and emotion outcomes. Frontiers in Psychology. 2019;10(967).
- Lee D, Hong SJ, Jung YC, Park J, Kim IY, Namkoong K. Altered heart rate variability during gaming in internet gaming disorder. Cyberpsychol Behav Soc Netw. 2018;21(4):259-67.
- Shaffer F, Ginsberg JP. An overview of heart rate variability metrics and norms. Frontiers in public health. 2017;5:258-.
- Rajakariar K, Koshy AN, Sajeev JK, Nair S, Roberts L, Teh AW. Accuracy of a smartwatch based single-lead electrocardiogram device in detection of atrial fibrillation. Heart. 2020;106(9):665-70.
- McArdle W, Katch F, Katch V. Exercise physiology : Energy, nutrition, and human performance. 6th ed. Philadelphia (USA): Lippincott Williams & Wilkins; 2007.
- Henley BC, Shokouhi M, Mahajan AY, Inan OT, Hajjar I. Cardiovascular response to mental stress in mild cognitive impairment and its association with cerebral perfusion. J Alzheimers Dis. 2018;63(2):645-54.
- Higginbotham MB, Morris KG, Williams RS, McHale PA, Coleman RE, Cobb FR. Regulation of stroke volume during submaximal and maximal upright exercise in normal man. Circ Res. 1986;58(2):281-91.
- Grassmann M, Vlemincx E, von Leupoldt A, Mittelstädt JM, Van den Bergh O. Respiratory changes in response to cognitive load: A systematic review. Neural plasticity. 2016;2016:8146809-.
- Scholey AB, Benson S, Sela-Venter S, Mackus M, Moss MC. Oxygen administration and acute human cognitive enhancement: Higher cognitive demand leads to a more rapid decay of transient hyperoxia. Journal of Cognitive Enhancement. 2020;4(1):94-9.
- Lenhardt R, Sessler DI. Estimation of mean body temperature from mean skin and core temperature. Anesthesiology. 2006;105(6):1117-21.
- Sawka MN, Cheuvront SN, Kenefick RW. High skin temperature and hypohydration impair aerobic performance. Experimental Physiology. 2012;97(3):327-32.
- Accuracy and precision of an accelerometer-based smartphone app designed to monitor and record angular movement over time. Telemedicine and e-Health. 2016;22(4):302-9.
- Izmailova ES, McLean IL, Hather G, Merberg D, Homsy J, Cantor M, et al. Continuous monitoring using a wearable device detects activity-induced heart rate changes after administration of amphetamine. Clin Transl Sci. 2019;12(6):677-86.
- Nair D, Tan SY, Gan HW, Lim SF, Tan J, Zhu M, et al. The use of ambulatory tonometric radial arterial wave capture to measure ambulatory blood pressure: The validation of a novel wrist-bound device in adults. Journal of Human Hypertension. 2008;22(3):220-2.
- Akintola AA, van de Pol V, Bimmel D, Maan AC, van Heemst D. Comparative analysis of the equivital eq02 lifemonitor with holter ambulatory ecg device for continuous measurement of ecg, heart rate, and heart rate variability: A validation study for precision and accuracy. Frontiers in Physiology. 2016;7(391).
- Smith CM, Chillrud SN, Jack DW, Kinney P, Yang Q, Layton AM. Laboratory validation of hexoskin biometric shirt at rest, submaximal exercise, and maximal exercise while riding a stationary bicycle. Journal of Occupational and Environmental Medicine. 2019;61(4):e104-e11.
- Flatt AA, Esco MR. Validity of the ithlete™ smart phone application for determining ultra-short-term heart rate variability. Journal of human kinetics. 2013;39:85-92.
- Hernando D, Garatachea N, Almeida R, Casajús JA, Bailón R. Validation of heart rate monitor polar rs800 for heart rate variability analysis during exercise. J Strength Cond Res. 2018;32(3):716-25.
- Raval NY, Squara P, Cleman M, Yalamanchili K, Winklmaier M, Burkhoff D. Multicenter evaluation of noninvasive cardiac output measurement by bioreactance technique. J Clin Monit Comput. 2008;22(2):113-9.
- Barr C. Comparison of accuracy and diagnostic validity of a novel non-invasive electrocardiographic monitoring device with a standard 3 lead holter monitor and an ecg patch over a 24 hours period. Journal of Cardiovascular Diseases & Diagnosis. 2019;7(5).
- Turvall E. Statistical summary: Study to evaluate the precision and accuracy of the oxitone 1000 when used in a continuous and spot prospective mode for non-invasive oxygen saturation measurements. 2017.
- Bilo G, Zorzi C, Ochoa Munera JE, Torlasco C, Giuli V, Parati G. Validation of the somnotouch-nibp noninvasive continuous blood pressure monitor according to the european society of hypertension international protocol revision 2010. Blood Press Monit. 2015;20(5):291-4.
- Bouillod A, Cassirame J, Bousson J, Sagawa Y, Tordi N. Accuracy of the suunto system for heart rate variability analysis during a tilt-test. 2015.
- Johnstone JA, Ford PA, Hughes G, Watson T, Garrett AT. Bioharness(™) multivariable monitoring device: Part. I: Validity. Journal of sports science & medicine. 2012;11(3):400-8.
- Rosenberg MA, Samuel M, Thosani A, Zimetbaum PJ. Use of a noninvasive continuous monitoring device in the management of atrial fibrillation: A pilot study. Pacing Clin Electrophysiol. 2013;36(3):328-33.
- Duan X. Electrocardiographic artifact due to a mobile phone mimicking ventricular tachycardia. Journal of Electrocardiology. 2014;47(3):333-4.
- Bent B, Goldstein BA, Kibbe WA, Dunn JP. Investigating sources of inaccuracy in wearable optical heart rate sensors. npj Digital Medicine. 2020;3(1):18.
- Bard DM, Joseph JI, van Helmond N. Cuff-less methods for blood pressure telemonitoring. Frontiers in Cardiovascular Medicine. 2019;6(40).
- Mehta Y, Arora D. Newer methods of cardiac output monitoring. World journal of cardiology. 2014;6(9):1022-9.
- Simić L, Riihijärvi J, Mähönen P, editors. Measurement study of ieee 802.11ac wi-fi performance in high density indoor deployments: Are wider channels always better? 2017 IEEE 18th International Symposium on A World of Wireless, Mobile and Multimedia Networks (WoWMoM); 2017 12-15 June 2017.
- Robinson R. Using biometrics as a social intervention in gaming. Proceedings of the Thirteenth International Conference on Tangible, Embedded, and Embodied Interaction; Tempe, Arizona, USA: Association for Computing Machinery; 2019. p. 755–60.
- Sousa A, Ahmad SL, Hassan T, Yuen K, Douris P, Zwibel H, et al. Physiological and cognitive functions following a discrete session of competitive esports gaming. Frontiers in Psychology. 2020;11(1030).
- Hessels RS, Benjamins JS, Cornelissen THW, Hooge ITC. A validation of automatically-generated areas-of-interest in videos of a face for eye-tracking research. Frontiers in Psychology. 2018;9(1367).
- Nagel GL. Use of eye tracking for esports analytics in a moba game: University of Bergen; 2016.
- T Fiori, A Auleear, I McIntyre, A Henson, R Dewan, T Gu, et al. Huum: White paper. 2019.
- Hoffmann CP, Filippeschi A, Ruffaldi E, Bardy BG. Energy management using virtual reality improves 2000-m rowing performance. Journal of Sports Sciences. 2014;32(6):501-9.