An observation of common physiological parameters during esports activity
by Aaron Koshy*, Lukas Cironis, Matthew McVey, Amber Sousa, Sophia L Ahmad, Tamzid Hassan, Kyle Yuen, Peter C. Douris , Hallie Zwibel, Joanne DiFrancisco-DonoghueEmail: a.koshy@nhs.net
Received: 14 Oct 2020 / Published: 26 Nov 2020
Abstract
Esports has become a significant international industry with more than 800 million people playing games from various esports titles every month. Physiological stress markers that are commonly utilised in other activities have not been adequately studied in esports. The current study examined changes in such stress markers during competitive gaming, at a cohort and team level. We also explore individual differences in respiration through the use of the Hexoskin suit. Increases in heart rate, respiratory rate and minute ventilation were found in all participants during an esports session when compared with a rest period. Notably, mean heart rate, respiratory rate, and minute ventilation were significantly higher in the winning team as opposed to the losing team (p<0.005). A high level of variance was also observed in between players across teams with respect to the respiratory rate. These results suggest significant cognitive workload and stress placed on players during competitive activity. With greater physiological understanding of the response to cognitive stress and teamwork during gaming, adaptations to training is likely lead to improvements in cognitive health, as well as individual and team performance.
Highlights
- Physiological monitoring during esports activity is feasible if a careful protocol is followed
- Common physiological parameters such as heart rate, respiratory rate and minute ventilation can vary significantly from rest to esports activity
- In this study, the winning team in League of Legends had larger increases in physiological parameters from baseline
- There appears to be a high level of physiological variance between players during play regardless of the game outcome
Background
Video games are a growing sector with an estimated 2.7 billion players globally [1]. This has helped generate significant value in esports, with over 800 million people playing popular titles on a monthly basis and a prize money total of $189 million across the top 10 games in 2019 [2, 3]. We define esports as competitive video gaming; two of the most prominent titles in this category are League of Legends (LoL) and Overwatch (OW). As esports grows with the rapid development of the professional scene in major esports titles, there would be an expected increase in investment made in players and team performance. Many major esports teams have coaching staff, data analysts and sports psychologists to support the players. Physical training and mental coaching are also often organised to improve performance further. Much of the rationale behind this high investment approach is based on improvements noted in traditional sports and feedback from the players themselves. Additionally, players often cohabit in gaming houses to optimise training through a controlled living environment. This has led to a need for objective assessment coupled with evidence that factors including stress and concentration can be derived from surrogate physiological markers, such as heart rate [4]. Indeed, during military, pilot or formula one training, biophysical tools are frequently utilised to derive additional performance assessment, as seen in both [5, 6]. We have previously noted the value of non-invasive monitors in esports and listed a range of commercially available tools [7].
Modern, non-invasive, biophysical assessment tools such as “smart clothing” include an array of sensors in a T-shirt-like garment. This enables the player to continue activity unimpeded whilst collecting data on parameters such as heart rate (HR), respiratory rate (RR), minute ventilation (MV), activity levels and heart rate variability [8]. Our team has previously reported the physiological and cognitive changes that occur in esports players prior to, during and following a discrete gaming session whilst wearing a smart shirt and performing mental tests [9]. This study extracted complete data from the smart shirt in the aforementioned study to look at the changes that occur in common physiological parameters during an esports session in greater detail. Specifically, we were interested in exploring the changes in mean HR, RR and MV during competitive gaming at a team level with further analysis of respiratory rate variation at an individual level.
The two games studied LoL and OW are team-based games in which players play as characters. Through the combination of mechanical skill, teamwork and strategy, defeat the opposing team of human players. LoL pits two teams of 5 against each other in which they draft a team of characters each with unique skills to defeat the opposing team through fights and strategic positioning with the overall aim of destroying the enemy base. OW games involve two teams of 6 players who choose heroes to play, each with their own set of gun mechanics and skills, with the goal being to defeat the opposing team through battles, and control of strategic points on the map.
Methodology
This study was approved by the New York Institute of Technology (NYIT) Internal Review Board. This work describes the physiological monitoring findings of an observational, well described, prospective study conducted by Sousa et al [9]. In summary, nineteen male esports players (part of the university esports team) signed written consent to take part in the study located at the NYIT esports arena, Old Westbury, NY, USA. Inclusion criteria allowed men or women between the ages of 18-30. Exclusion criteria included players who had not played competitively in the last year, were unable to wear the smart skin suit or had an injury that would affect their gameplay.
Following informed consent, smart shirts were fitted on the participants prior to competitive play. Specifically, participants wore the Hexoskin Smart Shirt® (5800 Denis St. Montreal, Quebec H2S-3L5). This garment is worn on skin and contains electrodes that enable measuring of common physiological parameters such as HR, RR and MV. Participants competing in LoL played in two consistent teams against each other, whilst OW players competed in cooperative teams of 2-4. Physiological parameter recording started upon placing the suit on the participants. After completing the esports session ranging from 1.5 to 2.5 hours across the two cohorts, participants removed the Hexoskin suit for data extraction.
Statistical analysis
Statistical analysis of the data was performed by author LC using R [10], a language and environment software for statistical computing. Recordings associated with HR and RR were accompanied with the quality measurements q hr /q rr respectively, which take non-negative values in ranges [0; 24] and [0; 15] respectively. Lower values of q hr /q rr correspond to the better quality of the observation. Based on the density and numerical values of q hr /q rr associated to HR/RR recordings, significantly poor-quality regions were omitted from the analysis. To account for the fact that data recordings are of different lengths, signal calibration techniques were applied to ensure that observations are in sync with each other before any comparison analyses were performed. In particular, maximisation of the autocorrelation among signals was used to detect the appropriate lag by which each signal needs to be adjusted. Changepoint analysis was performed to establish regions of rest and esports activity due to the absence of manual demarcations made during data acquisition. Deeper analysis of physiological parameter changes during activity was only viable in the LoL cohort as this took place in an appropriately controlled environment.
Finally, given the presence of multiple tests performed, Type I error was mitigated through conservative Bonferroni correction to adjust the significance level α=0.05. Given that for each biomarker the total number of sub-sessions is m=5, we test each sub-session at α/m = 0.01 level.
Results
In total 19 participants agreed to take part in the study, however 2 player datasets failed to transfer to the proprietary database resulting in analysis for 8 LoL and 9 OW players (Table 1). HR, RR and MV changed significantly during the esports session compared with the rest period (p<0.01) (Figure 1). Mean HR, RR and MV was significantly higher in the winning team as opposed to the losing team (p<0.01) (Figure 2). A high level of variance in RR was noticed between players across the two cohorts (Figure 3). None of the participants noted discomfort when wearing Hexoskin suit or believed that it impeded their play.
Discussion
We have noticed changes to common physiological parameters in players during esports activity in two mainstream titles, LoL and OW. Analysis of the combined cohorts of LoL and OW players show HR and RR increase during esport activity when compared with rest whereas MV slightly decreases. There is significant evidence that mental tasks or higher cognitive activity triggers a sympathetic response giving a rise to these physiological parameters [11, 12]. HR, RR and MV appear to vary significantly and frequently during the esports session. This likely represents the different game states that occur in both titles that can range from surveillance and preparation, to full blown team fights and individual outplays. Esports players are known to increase their HR during competitive sessions [13], however this is the first study to present findings of HR, RR and MV both continuously and statistically between sessions; giving greater insight into the multitude of physiological changes ongoing during play. This study found that in the LoL cohort, the HR, RR and MV was generally higher during gameplay in the winning team. This finding must be interpreted with care as it is observational and based on a small sample size, however, it is attractive to consider that this may be related to the winning team having a higher cognitive load or level of concentration, a factor associated with further increases in HR, RR and MV when performing the task [11, 12]. A similar response has been found in chess players. The high cognitive demands increase respiratory exchange ratio (RER) in this group further suggesting that physiological changes are occurring with higher cognitive activity [14]. Similarly, and perhaps counterintuitively, changes in physiological parameters seem to be persistent through the vast majority of the esports session, rather than simply at traditional key points in the game such as deciding team fights. In fact, significant variance in physiological parameters during play across games is sustained over hours in this study suggesting that these game titles require persistent concentration throughout the full course of the game. Cognitive tasks have been shown to cause a persistent rise in HR even with breaks between activity when compared to other actions such as listening to music [15]. This suggests that not only does partaking in esports produce a stress response from baseline, but that it likely fluctuates frequently during gameplay. Further investigation through synchronising data collection with recordings of gameplay may reveal more about this relationship. Similarly, our analysis of RR at an individual player level shows that there is a high level of uniqueness in the readings during gameplay. This is somewhat unsurprising as players in these games whilst working together as a team of 5 or 6, nearly always have exclusive roles and character positions at any one time. This means that there are a number of distinctive challenges they must face to succeed in the game, ranging from differences in environmental visibility around their character, to one on one battles with the enemy team. Another explanation is that there is a large diversity in individual response to cognitive tasks [11]. This implies additional value in creating a player specific strategy to their response profile during training via team level performance monitoring. Indeed, profiling of specific roles may be possible with further data collection, allowing role-specific characteristics to be determined for team player selection and subsequent tailored training. However, there are obvious points during the continuous measurement of the LoL players that presumably correlate with shared moments in the game such as the early preparatory stages or full team fights.
This study shows that it is both feasible and practical to conduct physiological monitoring in esports players. It is reassuring that none of the participants felt that their play was affected by wearing the Hexoskin suit. We note that two of the players within the LoL cohort could not be analysed due to issues around data capture with the Hexoskin suit. It is expected that these technologies will continue to develop at a rapid pace and soon allow for live monitoring to avoid such issues in future. This would be in keeping with live readings of HR found in smartphones or fitness trackers, despite the quality and accuracy of these devices being quite variable [16]. Physiological monitoring in general offers multiple benefits, such as objective measurements, continuous assessment during activity and the lack of reliance on the outward behavioural or physical response of the player. Greater collaboration with esports organisations, industry and healthcare professionals is expected to enable further research and performance understanding [7]. It should be possible to supplement the identification of areas of weakness in esports players, periods of stress and evaluate team communication via non- invasive assessment tools through future work. Indeed, our research suggests overlap between major esports genres in terms of physiological response during play, adding further value to the implementation of monitoring across teams. We believe that greater utilisation of technology within esports will not only significantly advance physiological understanding of the response to cognitive stress and teamwork, but also likely lead to improvements in individual and team performance, especially when assessment tools are used in combination with self-assessment questionnaires on performance or confidence. Improvements in performance may also be obtained from an increase in player confidence as a result of greater personal physiological understanding derived from combining physical and cognitive preparation. Improved training methods are likely to improve player awareness and confidence through an increase in their self-perceived control [17].
There are a number of limitations in this study. The sample size is small which made sub- analysis of individual roles within the game infeasible, despite the cohort size being similar to other esports studies. OW players conducted play over varying event sessions making it statistically impractical to produce a deeper assessment of variance in physiological parameters during play as produced with the LoL cohort. Furthermore, as the games were not recorded, it was not possible to clearly link key game states to physiological parameters which would have added further value to this study. As discussed, device limitations stopped assessment of two participants and also led to one LoL participant being removed from individual RR analysis. Variables that influence physiological parameters such as exercise, stimulant use, quality of sleep, physical health and emotional state were not accounted for. It is hoped that future work with monitoring devices is able to link physiological parameters to actions made by esports players in larger cohorts.
Conclusion
Esports activity across multiple game types results in significant increases to common physiological parameters from rest which appear to be both persistent and of varying intensity in players.
Figures
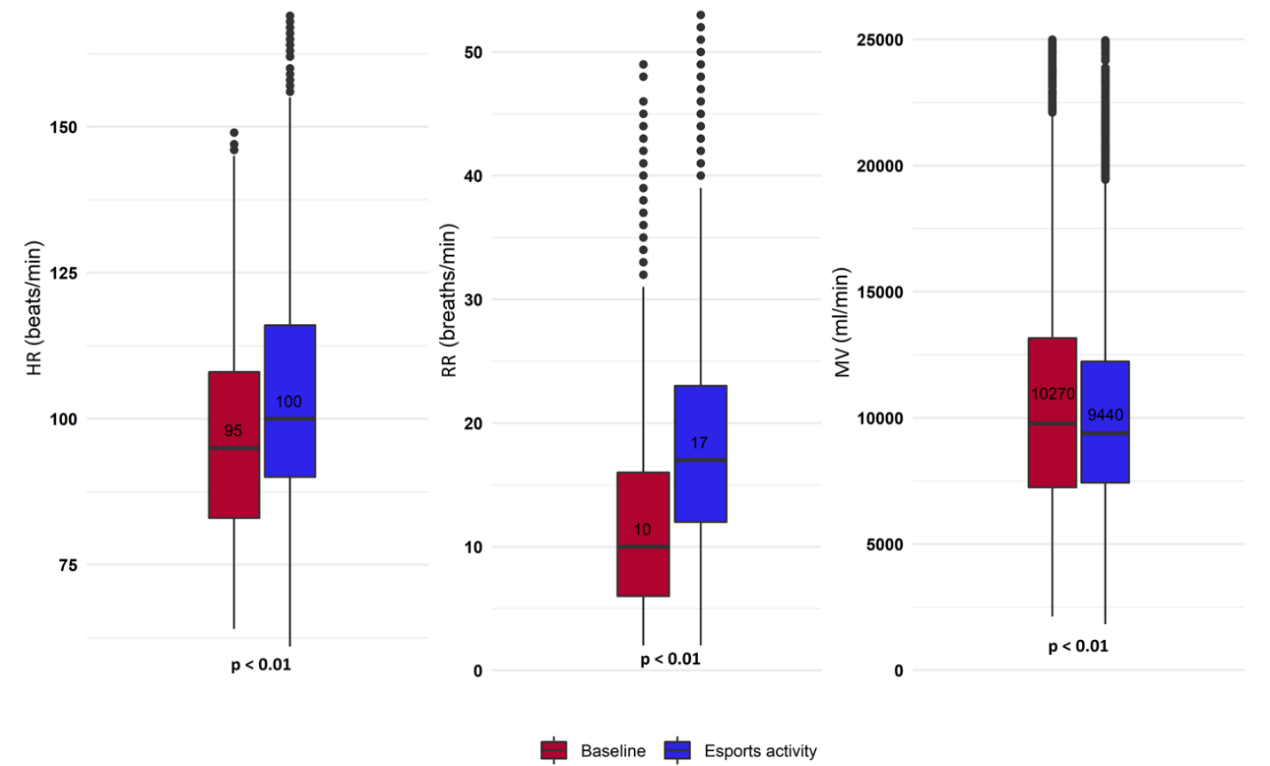
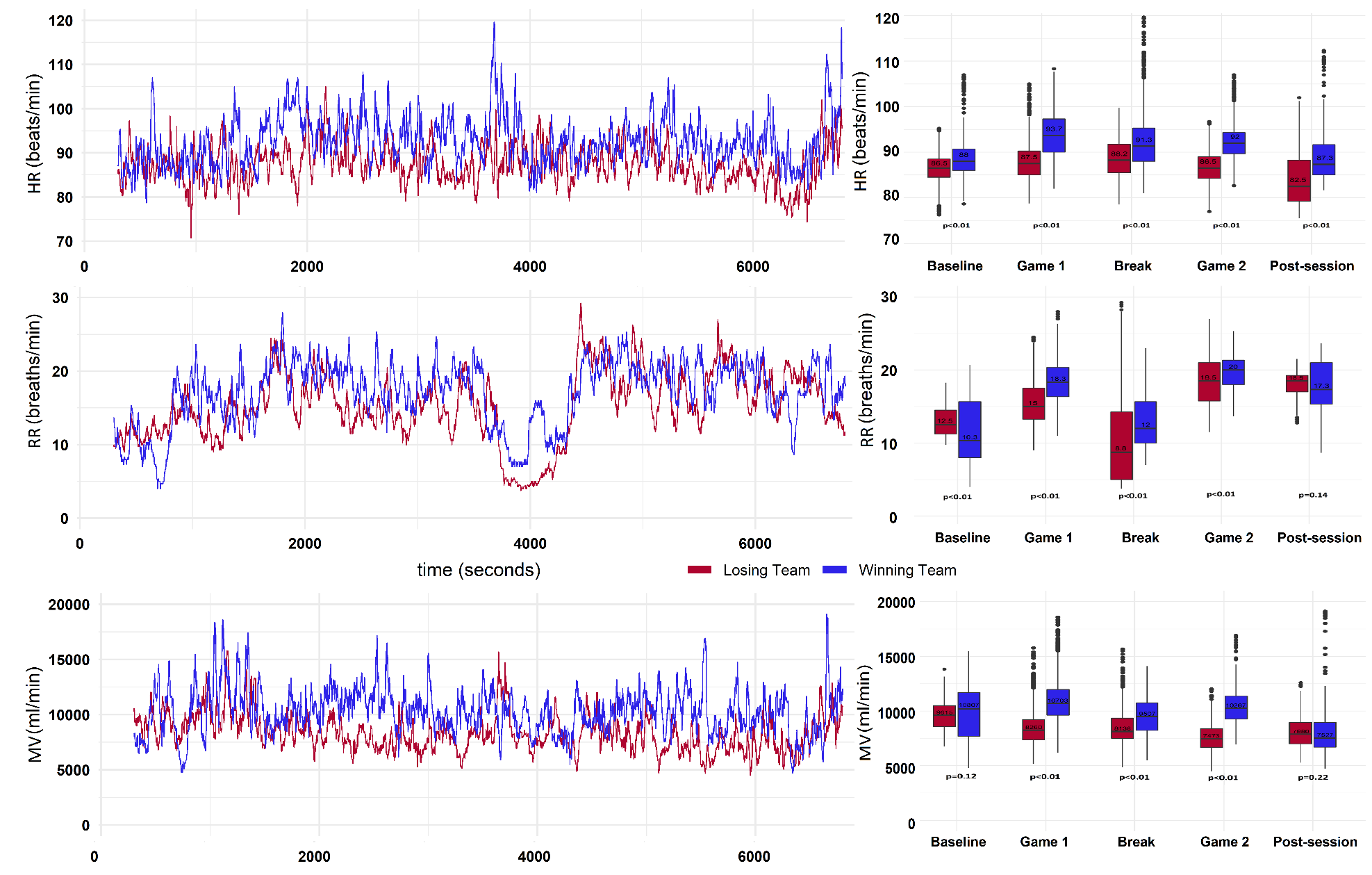
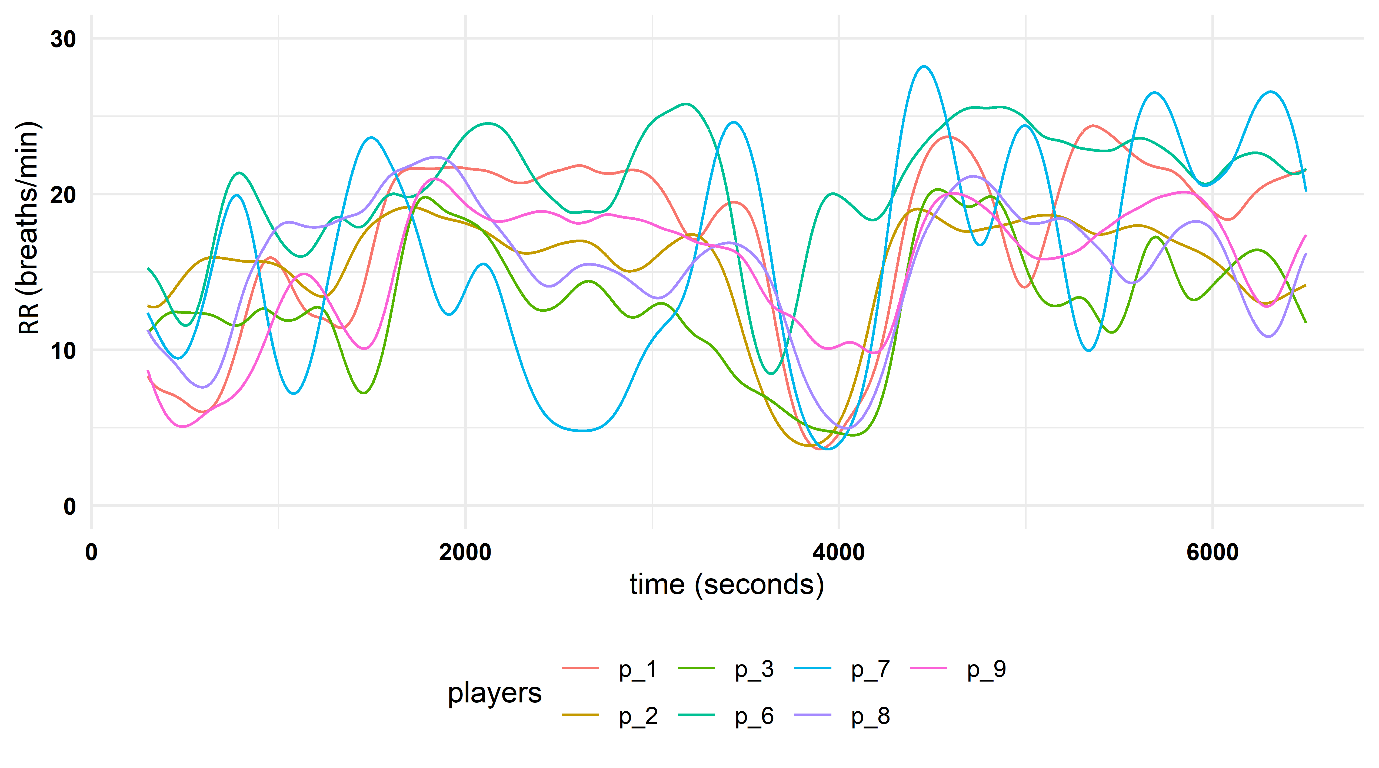
Tables
LoL players (n=8) | OW players (n=9) | ||
---|---|---|---|
Mean Age (years) | 20.8±2.1 | 19.4±1.3 | |
Male sex (%) | 8 (100%) | 9 (100%) | |
Winning team | Losing team | ||
Baseline HR (beats/min) | 86.0±9.1 | 88.6±7.1 | 77.9±10.4 |
Baseline systolic BP (mmHg) | 127±4 | 118±12 | 121±10 |
Baseline RR (breaths/min) | 15.3±0.7 | 13.7±3.5 | 15.7±4.6 |
Baseline MV (mL/min) | 12772.6±830.2 | 14192.6±4130.1 | 13434.8±3567.8 |
Median time spent playing casually (hours/week) | 3-4 | >6 | >6 |
Median time spent playing competitively (hours/week) | 1-2 | 3-4 | 3-4 |
References
- Newzoo. 2020 global games market report - april update. 2020.
- Wikipedia. List of video games by monthly active player count 2020. Available from: https://en.wikipedia.org/wiki/List_of_video_games_by_monthly_active_player_count#cite_note-8.
- Earnings E. Top games of 2019. 2019.
- Poulus D, Coulter TJ, Trotter MG, Polman R. Stress and coping in esports and the influence of mental toughness. Frontiers in Psychology. 2020;11(628).
- Friedl KE. Military applications of soldier physiological monitoring. Journal of Science and Medicine in Sport. 2018;21(11):1147-53.
- Brown J, Stanton N, Revell K. A review of the physical, psychological and psychophysiological effects of motorsport on drivers and their potential influences on cockpit interface design. 2019. p. 514-22.
- Koshy A, Koshy GM. The potential of physiological monitoring technologies in esports. International Journal of Esports. 2020;1(1).
- Smith CM, Chillrud SN, Jack DW, Kinney P, Yang Q, Layton AM. Laboratory validation of hexoskin biometric shirt at rest, submaximal exercise, and maximal exercise while riding a stationary bicycle. Journal of Occupational and Environmental Medicine. 2019;61(4):e104-e11.
- Sousa A, Ahmad SL, Hassan T, Yuen K, Douris P, Zwibel H, et al. Physiological and cognitive functions following a discrete session of competitive esports gaming. Frontiers in Psychology. 2020;11(1030).
- R core team. R: A language and environment for statistical computing. R Foundation for Statistical Computing, Vienna, Austria, 2017.
- Hughes AM, Hancock GM, Marlow SL, Stowers K, Salas E. Cardiac measures of cognitive workload: A meta-analysis. Human Factors. 2019;61(3):393-414.
- Grassmann M, Vlemincx E, von Leupoldt A, Mittelstädt JM, Van den Bergh O. Respiratory changes in response to cognitive load: A systematic review. Neural plasticity. 2016;2016:8146809-.
- Rudolf K, Grieben C, Achtzehn S, Froböse I, editors. Stress in esports - an insight into training and competition. eSport Conference "Professionalization of a Subculture?"; 2016; Bayreuth, Germany.
- Troubat N, Fargeas-Gluck MA, Tulppo M, Dugué B. The stress of chess players as a model to study the effects of psychological stimuli on physiological responses: An example of substrate oxidation and heart rate variability in man. Eur J Appl Physiol. 2009;105(3):343-9.
- Liu S, Kaufmann C, Labadie C, Ströhle A, Kuschpel MS, Garbusow M, et al. Short-term effects of video gaming on brain response during working memory performance. PLOS ONE. 2019;14(10):e0223666.
- Etiwy M, Akhrass Z, Gillinov L, Alashi A, Wang R, Blackburn G, et al. Accuracy of wearable heart rate monitors in cardiac rehabilitation. Cardiovascular diagnosis and therapy. 2019;9(3):262-71.
- Otten M. Choking vs. Clutch performance: A study of sport performance under pressure. Journal of sport & exercise psychology. 2009;31:583-601.